A vehicle’s character is defined by its suspensionsystem. The more tightly wheel suspension,tires, body, and driver interweave, the smoother your control over advanced driving maneuvers. For sporty drivers, this is often key to making a purchase decision. Of course, the chassis also has a major effect on driving safety and ride comfort. With electrified powertrains and the trend toward automated driving, the latter will be even more important in future than it is today. Disruptive progress, like the advances in e-mobility, is among the greatest challenges the international automotive industry faces. To address the greater requirements regarding driving dynamics and ride comfort, automobile manufacturers are seeking to employ advanced design tools.
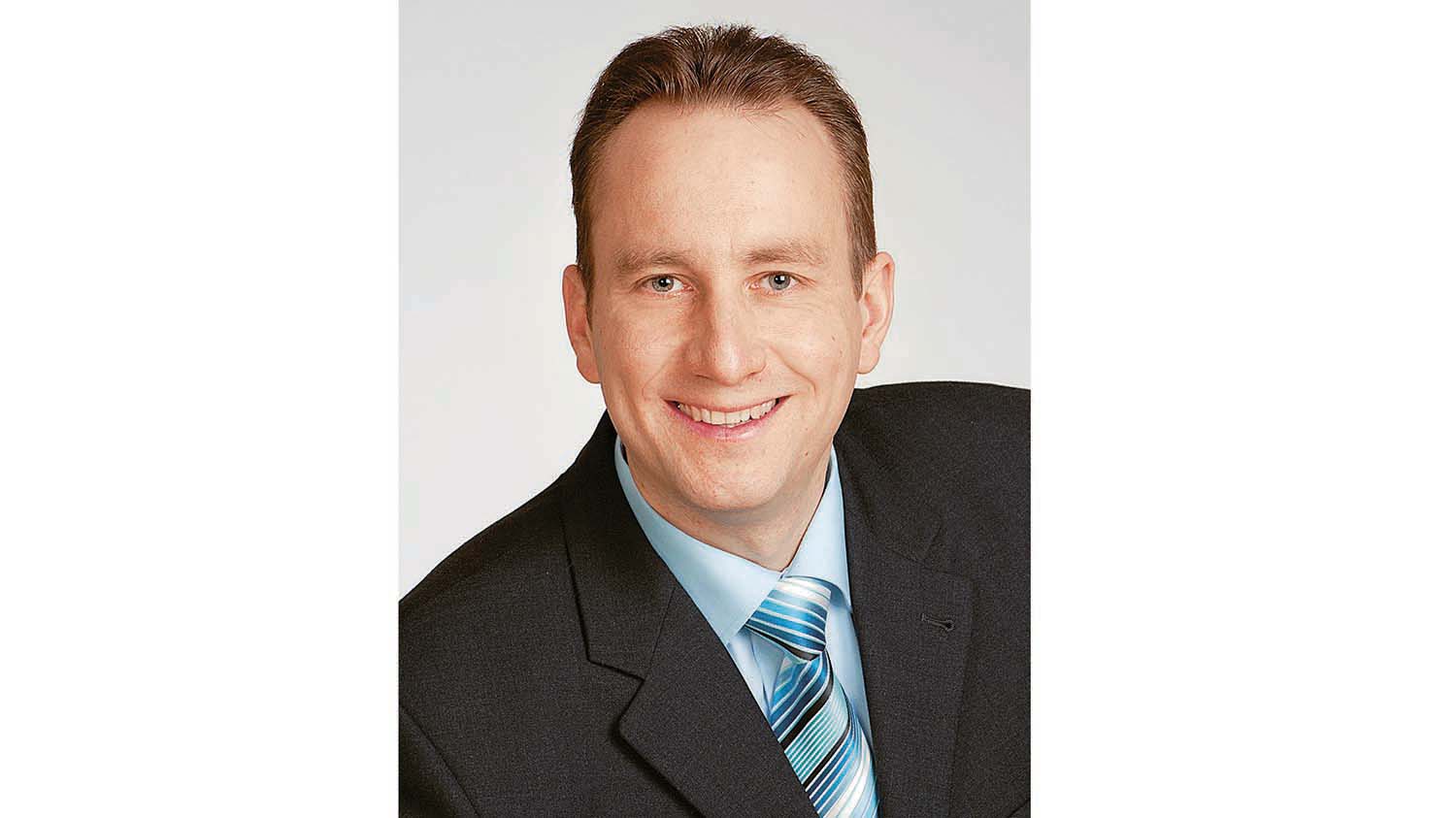
To understand the possibilities modern suspension systems offer, it’s worth taking a look at the sportscars Porsche developed in the past. Besides a driving dynamics control system stabilizing the vehicle in extreme driving maneuvers (Porsche Stability Management, PSM), they also feature smart torque distribution (Torque Vectoring). The variable shock absorber system PASM (Porsche Active Suspension Management) is another tried-and-tested element in this kind of semi-active suspension system. It responds to dynamic alterations, such as you might encounter during a sudden evasive maneuver, in the blink of an eye. In only fractions of a second, PASM ramps up shock absorber force on both axles to reduce lateral tilt or body see-sawing. Driving maneuvers of utmost precision become possible—and safe.
Falling back onto its experience with designing sportscar chassis, Porsche Engineering is able to offer its customers cutting-edge solutions. Like it did for a Chinese enterprise: “Our customer wanted us to design the front and rear axles for a model series comprising a number of electric vehicles from scratch and including the software algorithms and controllers for a variable shock absorber system,” says Johannes Wüst, Senior Manager Chassis Design. Dr. Martin Braun, Senior Manager Chassis Systems at Porsche Engineering, adds: “The customer specified benchmark vehicles we had to catch or outperform within a tight deadline and budget.” The goal was to build a suspension system that could hold its own against established international competitors when it came to ride comfort and driving dynamics.
"This kind of software-based control helps us keep production costs down.” Dr. Martin Braun
Though the front and rear axles’ mechanical design was conventional, getting it done in time to meet the tight deadline while also integrating the design specs of the overall platform was no minor feat. One of these specs, for example, was a double wishbone axle with alloy arms and pivot bearings. The lower control-arm plane was resolved to optimize scrub radius and Ackermann toe-out. “For the rear axle, we focused heavily on getting a really good shock absorber ratio and longitudinal compliance,” Wüst explains with respect to the 5-arm design. “We also aimed for excellent elasto-kinematics and rigidity in both axles.” The resulting axle designs are now suitable for both sedans and SUVs in terms of spring travel, maximum axle load, and fatigue limits.
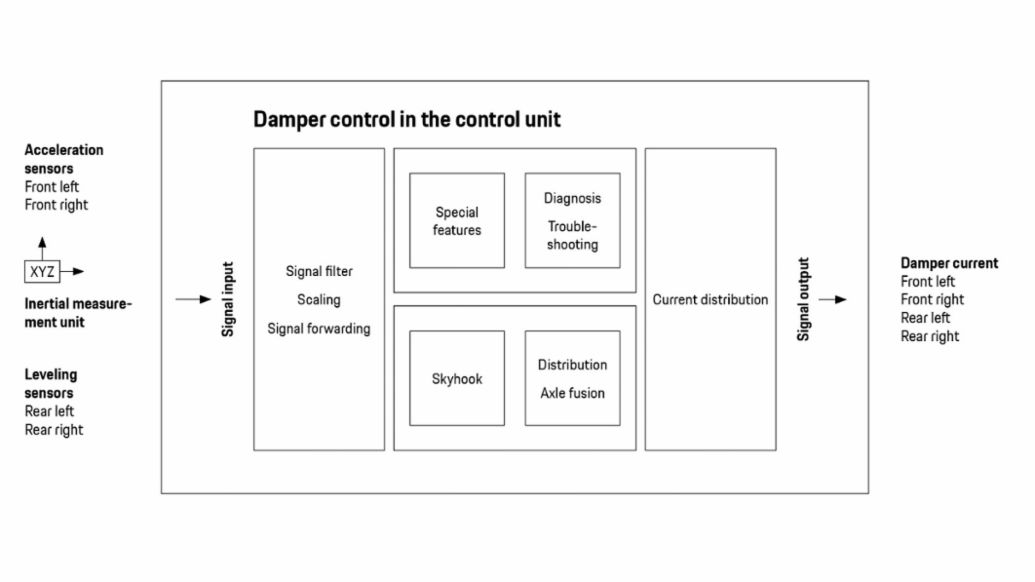
To obtain the specified driving characteristics, the engineers developed a special electronic system architecture for the front and rear axles’ damper control system. This system architecture allows the damper controller to adjust perfectly to the control systems in the respective vehicle. On top of that, it supports a variety of sensor layouts, in turn making custom-tailored solutions feasible in short times at little expense. “This kind of software-based control is complex,” Braun says. “But it helps us keep production costs down by letting algorithms handle the lion’s share of comfort and driving dynamics functions.”
The skyhook function keeps the vehicle body as steady as possible
In the end, this meant using only a small number of sensors and actuators. Instead of four leveling and eight acceleration sensors complementing an inertial measurement unit (IMU), the IMU now interacts with only two acceleration and two leveling sensors. The result is the cost-optimized, smart damper control system (Continuous Damping Control, CDC). The system expands upon the familiar skyhook feature with a whole range of software-based add-ons—among them pothole detection and lane changing.
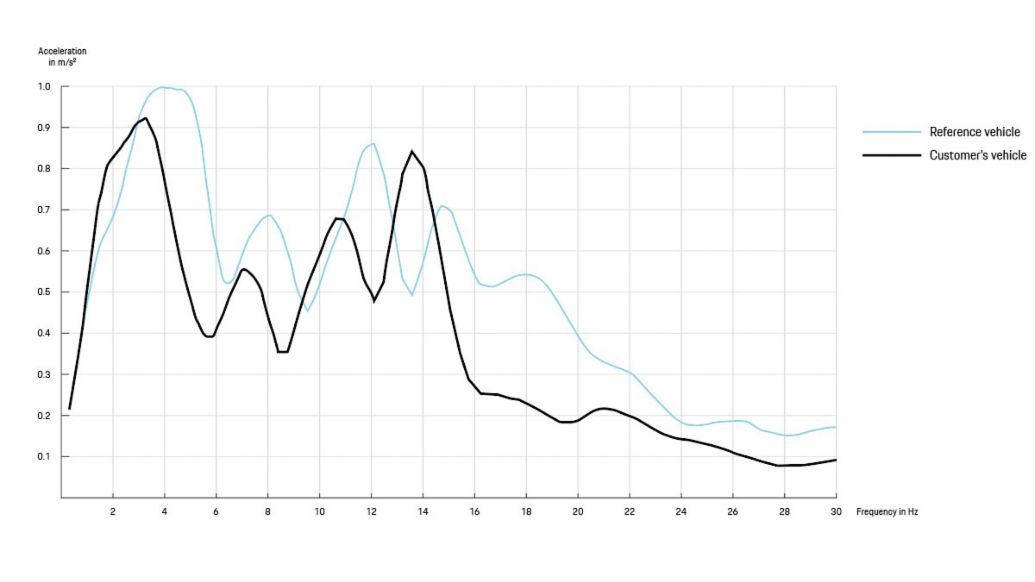
When active, it keeps the vehicle body as steady as possible, irrespective of the present road conditions, almost as if the vehicle were actually attached to skyhooks. While driving, the control system manages to damp or neutralize vibrations across the entire frequency range, especially those in the higher-frequency range from 16 to 30 hertz.
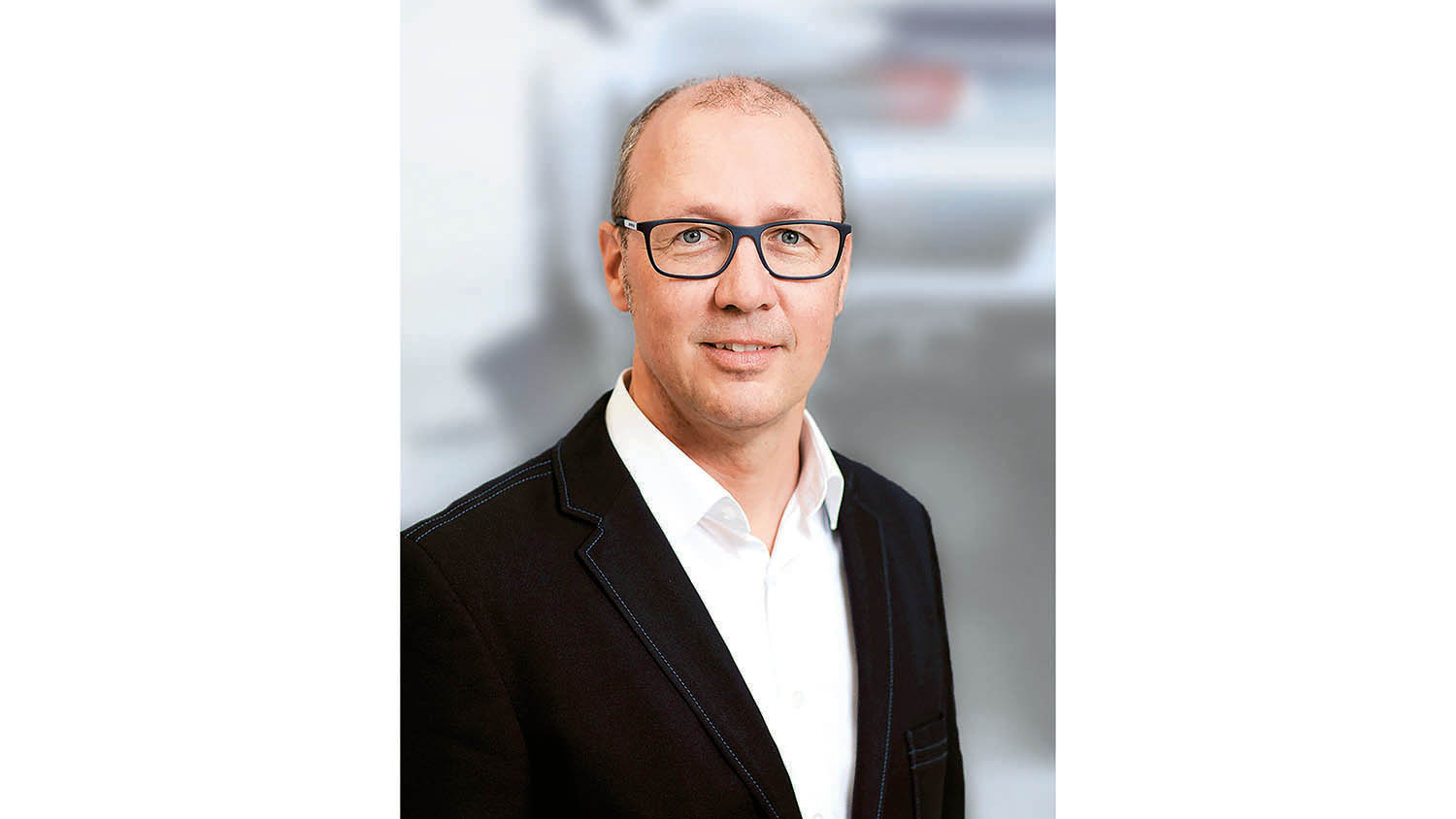
Besides first-grade technology solutions, the Chinese OEM also expected close collaboration and support in setting up their capacities. “To begin with, our customer’s R&D team was still quite small at less than 400 staff. It grew considerably over the course of the project,” Wüst remembers. “An entirely new production infrastructure was set up, alongside designing the vehicle itself.” Working closely with the customer’s production division, Porsche Engineering’s engineers discussed the requirements mass production needed to meet and incorporated the findings in their designs. Experience gained in past projects and on Porsche’s production lines in Zuffenhausen and Leipzig proved invaluable, too.
One of the greatest challenges was the customer’s tight schedule. Start of production was expected only 18 months after completing the first prototype. To meet this specification, Porsche Engineering began using simulation platforms and rapid prototyping system right from the beginning. “This let us get the first prototypes running for tests really quickly,” Braun elaborates. The specifically designed mule vehicles make it possible to quickly draft and finetune an axle concept in the early design phase. “This way, we can avoid major change cycles during the trial phase,” says Wüst. “So we’re not only saving on development time, but also cutting down on test components and prototyping tools and ultimately saving a lot of money.”
Another benefit was that working simultaneously on the control system and on the software offered extra potential for optimizing. Wüst is full of praise for the Chinese customer here, lauding that the design targets and vehicle variants were already defined with great precision when the project kicked off.
“An entirely new production infrastructure was set up, alongside designing the vehicle itself.” Johannes Wüst
During the project itself, decisions on where to take things were always reached quickly and pragmatically, every time taking into account Porsche Engineering’s advice and avoiding delays. “Without these rapid-fire decisions, the short development schedule would have been our undoing,” Wüst confides. In the end, the ambitious schedule was met and the model series went into mass production according to plan in March, 2020.
Info
Text: Andreas Burkert
Contributors: Dr. Martin Braun, Johannes Wüst
Text published in Porsche Engineering Magazine, No. 1/2020