High energy content, high performance, long service life, maximum safety—all at the lowest possible cost: Batteries in electric vehicles have to meet many requirements, which the dominant lithium-ion technology is already succeeding at quite well. Further improvements can, however, be made to almost all of its parameters, and researchers and industry are currently working intensively on doing just that. At the same time, potential successors are already lining up. It is no coincidence that lithium-ion batteries dominate today’s market: Lithium atoms are particularly keen to emit one of their three electrons, and lithium is also the lightest metal. This makes the element a popular raw material for batteries.
“Pure lithium is the ideal active anode material in terms of energy density,” says Dr. Stefanie Edelberg, Specialist Engineer Battery Cell at Porsche Engineering. “For safety reasons, however, graphites are currently used primarily as active anode materials that can absorb lithium ions.” In addition, the charging capacity of the batteries is very high and their price is relatively low. Added to this is their long service life: “1,500 to 3,000 full charge cycles until a residual capacity of 80 percent is reached does not present a problem,” says Dr. Falko Schappacher, Commercial and Technical Director of MEET Battery Research Center at the University of Münster (WWU). Car battery service lives of up to one million kilometers are now being predicted.
Anode optimization
Because lithium-ion technology is a multi-component system, there are many ways to optimize it further. Take, for example, the anode: Graphite is currently used as an active anode material. Silicon is an interesting alternative to this because it offers a storage capacity that is ten times higher. “Silicon anodes would significantly increase the total capacity of the lithium-ion battery,” as Schappacher underscores. Edelberg also points out the advantages: “Silicon is of particular interest because it exhibits the second-highest storage capacity in terms of weight after lithium, which allows for cells with very high energy densities. What’s more, it is the second most common element in the earth’s crust.” Cells with a high fast-charging capability and which can be charged from five to 80 percent in less than 15 minutes are indeed feasible.
“However, when lithium is absorbed, the silicon particles expand by 300 percent, resulting in mechanical stress in the material and electrode,” says Schappacher. If this were to damage the electrode surfaces, the service life of the battery would also be impaired. “The biggest leverage in terms of energy density is attained by using pure silicon active material, but then you also have to contend with the worst downsides in terms of service life,” says Edelberg. Nevertheless, intensive work is being carried out on anodes with a very high proportion of silicon of up to 80 percent. This is the path that Cellforce (see box), for example, is following in conjunction with Porsche.
More nickel in the cathode
Intensive work is also underway on optimizing the active materials for the cathode. The important thing in this case is a combination of a large charging capacity and a high electrochemical potential of the material. At present, lithium-nickel-cobalt-manganese-oxide (NCM) in a ratio of 6:2:2—in terms of the parts nickel, cobalt, and manganese—is most frequently used in electromobility in Europe.
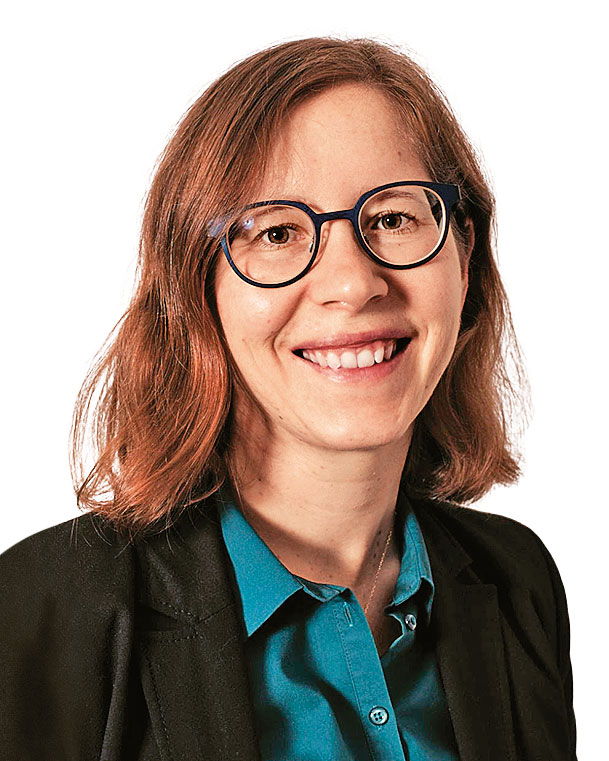
In the future, nickel’s share is likely to increase, while cobalt and manganese will be used to a lesser extent. The growing share accounted for by nickel promises higher charging capacities. Further optimization potential is offered by the separator, which consists of very thin (10 to 20- micrometer) films, mostly comprised of polyethylene or polypropylene. This separator costs space and weight. “The separator can indirectly contribute to the energy content of a battery cell,” says Edelberg. “The thinner it is, the more layers or coils on electrodes fit into a cell. This increases the cell capacity and the energy content of a battery cell.”
Compact solid-state batteries
Solid batteries, an area into which intensive research is being conducted, could need significantly less installation space than conventional lithium-ion batteries. They do not use an electrolyte solution, but a solid supporting electrolyte. “The plan for solid cells is that the classic separator will be completely replaced by a thin layer of solid electrolytes,” explains Edelberg. “The solid electrolyte is then both electrolyte and separator in one.”
“The biggest leverage is attained by using pure silicon active material.” Dr. Stefanie Edelberg, Specialist Engineer Battery Cell at Porsche Engineering
By eliminating electrolyte solutions and using lithium-metal anodes at the same time, researchers hope to achieve an increase in energy density of up to 50 percent, and possibly significantly faster charging times as well as a low flammability of the solid electrolyte.
By eliminating electrolyte solutions and using lithium-metal anodes at the same time, researchers hope to achieve an increase in energy density of up to 50 percent, and possibly significantly faster charging times as well as a low flammability of the solid electrolyte. Compared to other developments such as lithium-air batteries, Schappacher sees lithium-based solid-state batteries (SSB) as “a serious alternative to lithium-ion batteries”. Sodium-ion batteries (see box) are of particular interest for local storage applications due to their lower energy density. Lithium-air technology still poses many challenges and, as things stand, promises very few advantages. “Currently and for the foreseeable future as well, lithium-air cells are definitely still a topic for basic research,” says Edelberg. Cell chemistry, however, is not the only way to optimize batteries. Cell sensors and packaging offer further potential. Battery charge levels, for one, can be detected more precisely and quickly by sensors in the cells. This allows the charging time to be shortened—for example, by allowing fast charging in special voltage ranges. Cell cooling can also be controlled more precisely, which is a boon to battery longevity.
In the future, packaging and cell design will also play a major role in making batteries more powerful. Cell-to-pack technology, for example, integrates the cells directly into the battery pack. “This eliminates the small-scale parts in current batteries,” says Prof. Maximilian Fichtner, Director of the Helmholtz Institute Ulm (HIU) and Head of the Energy Storage Systems research unit at the Karlsruhe Institute of Technology (KIT).
“Instead of connecting chocolate-bar-sized cells individually, cells up to 1.20 meters in length are now tightly packed when installed crosswise into a frame, just like the slatted frame of a bed.” This results in more storage capacity and better cooling in less space.
Further potential
“In the medium term, we can expect the combination of new anode chemistry and dense packaging of the cells to allow a vehicle range of 1,300 km,” says Fichtner. Schappacher is also optimistic—even if it is difficult to predict the impact of technological advances such as the solid-state battery. “I think that we will see 30 to 50 percent increases in range in premium vehicles in the future,” the expert expects, stressing: “More important than simply increasing the range is the fast-charging capability.” Schappacher expects that, one day, fast charging to 80 percent of the vehicle’s range won’t take much longer than a fuel stop.
“In today’s Taycan, a charging time of 22.5 minutes was able to be achieved when charging from five to 80 percent,” explains Markus Gräf, Chief Operating Officer of the Cellforce Group (also see box). “With silicon as an anode material, values of less than 15 minutes can be attained in the medium term, and significantly lower ones in the longer term.” This said, new and more powerful charging stations would also have to be developed for this purpose. In addition, charging sockets will need active cooling in the future so that high charging capacities of more than 500 kW can be reliably conducted. Optimized lithium-ion batteries and new technologies such as solid state batteries: Thanks to intensive research and development, electrical energy storage systems are set to become much more efficient in the coming years—making electric mobility even more attractive.
Info
Text first published in the Porsche Engineering Magazine, issue 1/2023
Text: Chris Löwer
Copyright: All images, videos and audio files published in this article are subject to copyright. Reproduction in whole or in part is not permitted without the written consent of Dr. Ing. h.c. F. Porsche AG. Please contact newsroom@porsche.com for further information.